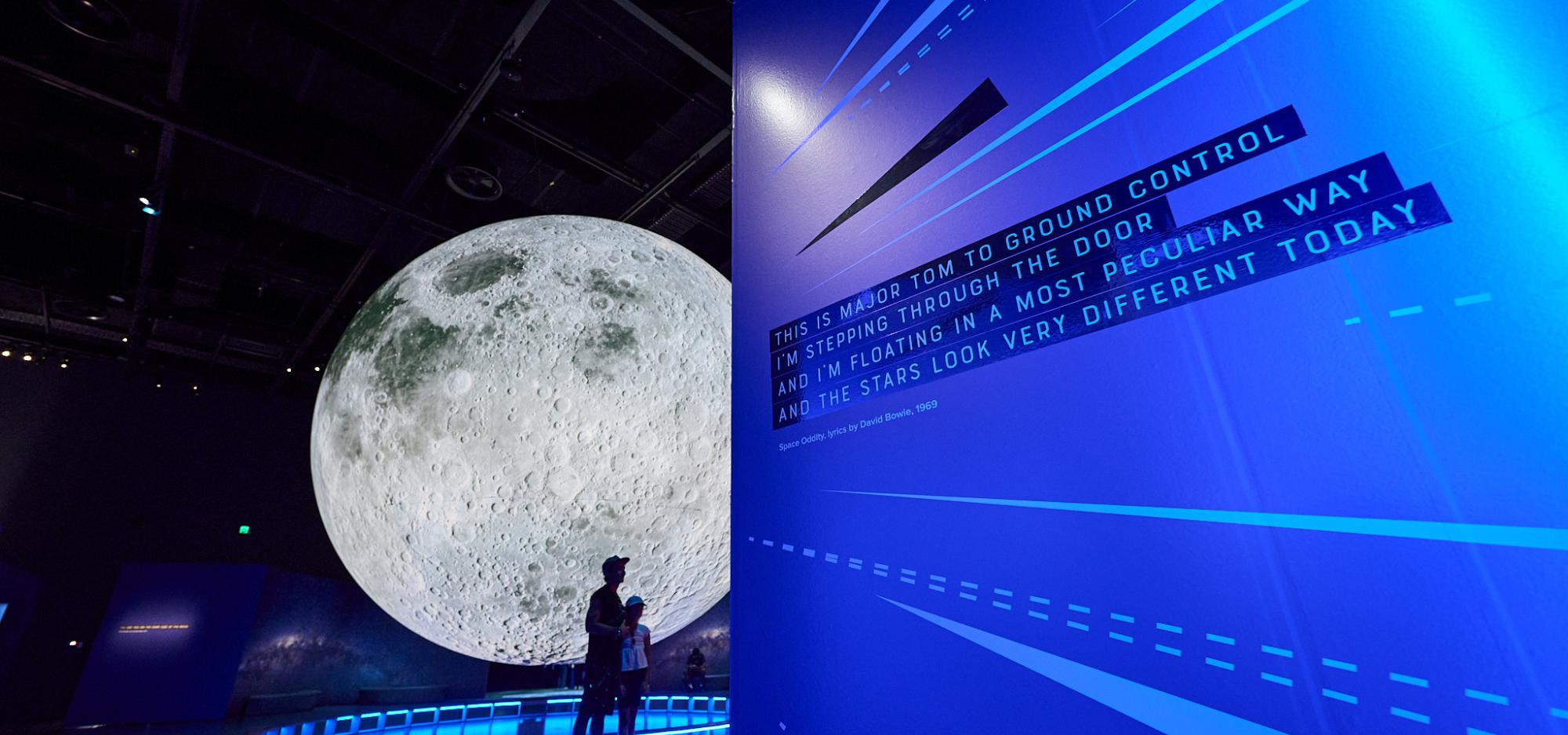
Multi-messenger Astronomy and the New Era of Cosmic Exploration with Professor Eric Howell.
Join Professor Eric Howell in this talk that delves into the heart of multi-messenger astronomy, highlighting its potential to decipher the intricate mechanisms driving these cosmic phenomena.
In the vast expanse of the universe, cosmic phenomena like gamma-ray bursts emit signals across multiple messengers - from electromagnetic waves to gravitational waves, offering us a unique window into the most energetic and enigmatic events in the cosmos.
The field of multi-messenger astronomy uses these diverse signals to unveil the mysteries of the universe's most violent processes.
It will introduce the topic from the groundbreaking joint observation of a gravitational wave event and a gamma-ray burst in 2017 through to the launch of a new gamma-ray satellite, MoonBEAM, dedicated to multi-messenger astronomy and positioned at a strategic Earth-Moon Lagrange point.
This talk was recorded as a part of the Lunar Lounge series for the To The Moon exhibition.
-
Episode transcript
Transcript coming soon.
More Episodes
Join Dr Robin Cook on a celestial journey exploring the mesmerising interplay of the Sun, Moon, and Earth
In this talk Professor Bland outlines the mission that Curtin is developing to find ice on the Moon in accessible locations.
Join Xavier de Kestelier, Principal and Head of Design at Hassell, to hear about Hassell’s design journey and collaboration with the European Space Agency to develop a innovative concept for a thriving settlement on the lunar surface.
Join Dr Benjamin Kaebe, an engineer, researcher and teacher, as he discusses the opportunities of a Western Australian space economy.
Professor Phil Bland provided an overview of Artemis, and what the next decade of space exploration might look like.
In this lecture, Professor Katarina Miljkovic explores the impact origin of the Moon.