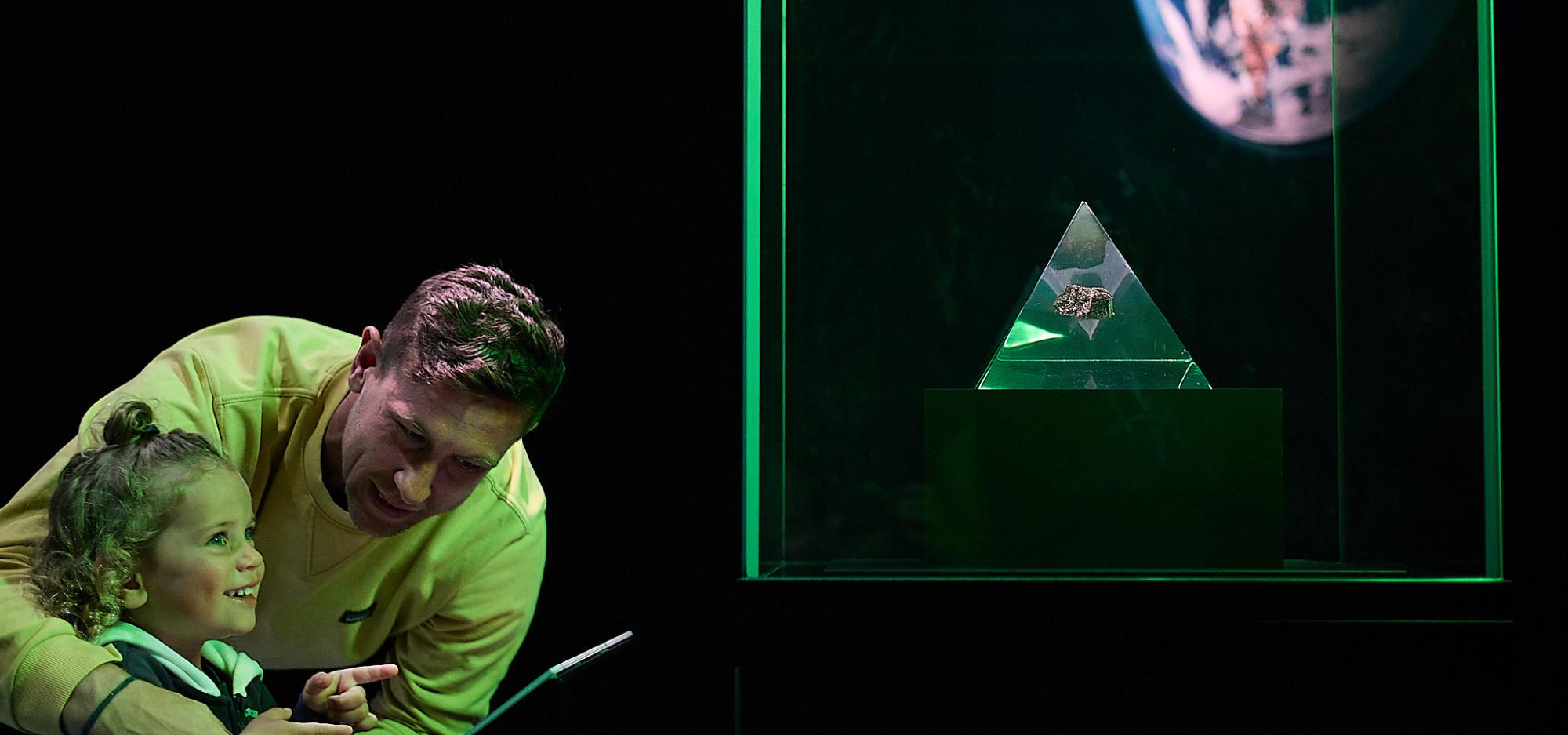
Engineering Plants for Space Exploration with Dr Adil Khan
Explore the realm of off-earth habitation and hear how plants can be engineered for space exploration and altered Earth climates.
Dr Adil Khan discusses a recently developed new programming language that can be written into a plant’s genome to improve agricultural yields and adapt crops for distinct environments.
-
Episode transcript
[Recording] You're listening to the Western Australian Museum Boola Bardip talks archive. The WA Museum Boola Bardip hosts a series of thought-provoking talks and conversations tackling big issues, questions and ideas, and is delighted to be able to share these with you through the talks archive. The talks archive is recorded on Whadjuk Nyoongar Boodjar. The Western Australian Museum acknowledges and respects the traditional owners of their ancestral lands, waters and skies.
MC: Good evening everybody and welcome to the WA Museum Boola Bardip and this Lunar Lounge event at the To the Moon exhibition. Before we get started with our guest speaker tonight, I would just like to take a moment to acknowledge the lands on which we are gathered and learning on here tonight, the lands of the Whadjuk People of the Nyoongar nation. May we pay our respects to elders, past and present, and extend those respects to any First Nations folks joining us tonight.
This evening we're joined by Doctor Adil Khan, who over the next 30 minutes will be taking us through Engineering planets for space exploration. Please make them feel welcome.
Adil Khan: Thank you very much. First of all, thanks to the WA Museum for giving me the opportunity to present our work here. Tonight I am going to talk about the technology that we have developed, and we are using it to program plants for space. And I work here at the University of Western Australia at the ARC Center of Excellence in Plants for Space.
So before I go into the details of this technology that how we are programing plants for space, I would like to talk a bit about our center. ARC Center of Excellence in Plants for Space was established earlier this year, January. And the aim of this center is to program plants for space, making the plants to adapt to the unique space environment for space exploration and habitation in the near future. So our center has four different programs which quite overlap with each other. So the first one is engineering plants for efficient growth, minimal waste, on demand material and medicine. The second program is to improve processing, structure and texture of the plants so that it is easier for the extraction and purification of materials and medicine. Program three is about further enhancing the plant nutrition, flavor and storage. And program four is about training the next generation about the technologies that are developed at the center. So tonight I will cover some part of the program one, that how we can engineer plants for efficient growth and on demand production of different materials and medicine. So, and this is quite important not only for the space exploration but also there are some issues on the Earth that could be addressed with these technologies. So, we need new technologies to improve agriculture. As you can see here, with this rate of the UN biodiversity, that 80% of the food that we eat today is coming from plants, and then 40% of the food crops are usually lost per year due to plant pests and diseases every year. And of course, the world population is increasing so we need better plants to meet the global food requirements. Now, if we look into the history that how plants were improved. So they used to do, many, many years ago like those enough years ago, selective plant breeding. And for example, on the left hand side, you can see here we have a plant variety which is high yielding but it is disease susceptible. And then they used to cross it with another variety, for example, that is not very highly high yielding but disease resistant. And through many, many cycles of crossing then used to get elite variety with disease resistance. If I give you an example here, so that would be [unknown species name] which is a small bush plant, was found in Mexico and around 10,000 years ago. Like, I mean, this crossing started and today we have this modern corn here. This is, this was done, like, in the traditional way. But then in the early 1920s what happened is people, the scientists, they started taking foreign genes and then introducing them into the plants to improve their performance. That could be for yield, against disease resistance. It reduced the amount of time it was taking from thousands of years to 6 or 7 or 8 years and maturity of this kind of engineering has been done to improve growth in yield, resistance against drought, heat, resistance against bacteria.
So this foreign gene that is introduced into the plants, taking it from other species, it is switched on all the time and you can resemble a tube just like the light switch. This bulb, when you switch it on, it is switched on. But if you leave it on, for extended period of time, so there is a cost for this because your electricity bills will be then quite high. So, same is the case with the plants, or any living organism, if you introduce any foreign gene and then that is left switched on forever. So then it leads to metabolic burden on the plant performance silencing deleterious. So what we have done, we have reduced the amount of time for engineering plants. But at the same time it is not perfect. Wouldn't be good if we can engineer smart, smart plants where they can integrate multiple input signals that could be environmental. For example, any chemicals, any heat signals, or any bacteria, anything you can think of and then you program the plants in a way that when and where you need it to produce a response.
So it is possible with the help of the technology that we are working on called gene circuits. So you can think of the gene circuits similar to the electronics circuit. So here is just an example of the light switch. When you want to switch on you can switch on, when you want you can switch it off. On the other hand the gene circuits they are composed of DNA, RNA and proteins. I will give you a very simple example here on the right hand side. So it is a simple gene circuit which is composed of gene and these genes incurred then proteins. And so here I have this example of the luciferase, luciferase reporter is just a protein that you can visualize. So sticking to the example of this reporter gene in ideal condition, when you introduce it in plants it will remain switch on. However, when you have an input signal in the form of a repressive, for example, here we have this dcas9 [unclear word] system. When you target this gene, you can see here that protein won't be produced. So it is just like a switch. So on the left hand side it is on, on the right hand side when you have this designer protein it is switched off.
So this is something we were working on to introduce in plants. But now the question is, how can we test it in plants because our plants they have long life cycles. It takes many, many years. What if there is some problem with this record? How we can detect it? So for this purpose, what we do, we take plant leaf and then we extract the cells from the leaf. And then we test the circuits in there to test the performance, whether the circuit is functional in plants. So, in using this assay we can get our results within 24 hours. So instead of many, many years within 24 hours. Like we do the experiment today, the very next day we have the results.
Sticking to that example of the reporter gene. So we established this system in the lab here and we tested it in plant cells. So here you can see you don't have to go into all those molecular details, but I just want to show you that when we don't have the input signal here, the green bar, it shows that the switch is on. However, in different combinations of the input signals it is switched off. So we had successfully demonstrated it. It is just one slide here. But all the optimization and all the experiments, it took us three years that we were able to show it, but we got to that point.
Now you might be thinking because the problem we have is with the real plants, and all I'm showing here is just the plant cells. So that was the next challenge. I'm coming back to my previous slide that we are after a technology that we can introduce into the plants, which can integrate multiple input signals, and then it can produce a response like a desired response when and where we want it. So for this purpose, what we did, we introduce our circuit into the plant and we linked it to the environmental signal. That is just the heat treatment, to see whether it is functional in real plants. So for this purpose we took the model plant Arabidopsis, which is quite small, and if you want to transform it and get the results it takes like up to almost nine months. So here I'm going to show you some of the results that how this system is working.
So we tested the performance of our circuit when we exposed the plant to the heat. And we can see in the cotyledon, the cotyledon these are small like the initial leaves which the plant emerge during the growth. So here you can see when we don't have the heat treatment the circuit is switched on. And when we have the heat treatment the circuit is switched off.
Then we tested it in plant roots. Here you can see again that the circuit is on in the absence of any input heat signal. And it is switched off in the presence of the heat signal. And then, because to date, whatever people had done previously when they introduced a circuit into the plant, so it used to switch on forever. So for example they could link it to any environmental signal. But the problem that used to be it remained switched on after exposure to that environmental signal. So what we had done because we were after something that could be reversible. And here I'll show you this is the data from the plant leaves. What we did there, we exposed the plant leaves, the real plant to the heat signal at 37°C. And you can see that at zero hours the circuit is switched on. And after six hours you can see that the circuit is, the gene is switched off. And then after 24 hours, it can start to revert back to the original state. And then the circuit is fully reverted after 48 and 72 hours. So, we could successfully demonstrate that these circuits that we have developed, they are highly programable because we could link them to the environmental signal.
And then here this data suggests that they are reversible. Now, to be honest, it took us nine years to get there because this is the first time that somebody has done it, they were reverted. For the first time we could create a circuit that is highly programable and then it is reversible. So now, because all of this data, this is coming from some model organism, Arabidopsis, the question would be because whether this one is functional in different plant species or not.
So I'm going to show you some of the preliminary data here, which is Physcomitrella patens, which is moss. So we have tested the performance of this circuit in the cells of Physcomitrella and you can see in the absence of the signal the circuit is switched on, in the presence of the signal the circuit is switched off. We also tested it in the cells isolated from wheat and the results are promising. In the absence of the signal the circuit is switched on in the presence of the signal the circuit is switched off. We also tested this one in Brassica and you can see that the circuit is switched on in the absence of the signal. And then in the presence of the signal it is switched off. So all this showed that now we are capable of reprograming plants. And to summarize this one that we can use these synthetic circuits to program plants, not only model organisms, but also different cross species. And we can specifically turn on useful genes on and off. So I think we are ready now that we can write the genomes and then programing plants for nutrition, flavor, storage, material and medicines and different characteristics.
I think I have got a few minutes, but I just want to show you some other data. If somebody is here from computer science, electronics, so they would know where these logic gates are because these are the engineering principles which you can dictate, like condition specific. They are mainly used for controlling the flow of current. But here, I’ll use this one to demonstrate that we can also, the simple circuit that I just showed here, that we can layer them together to perform complex computations. For example, I have here imply B be like in different combinations being imply A and gates. So that is different combinations and we can successfully program plants.
So this is some of the work that we do at the ARC Center of Excellence Plants for Space. And this would not have been possible without highly talented team. Some of the team members are also present here. So I would like to pay great thanks to my mentors, Ryan Lester, the rest of the lab, the ARC Centre of Excellence for Plants in Space, the University of Western Australia, ARC Center of Excellence in Plants, Plant Energy Biology, CSIRO and the Australian Government. Yeah. [Applause]
MC: Wonderful, thank you so much for that. We've got time for a few questions if anyone wanted to ask a question at all. Anyone at the back? Oh yes, great. I'll come to you with mic.
Audience Question : Hello. So if I understood, so you say you're able to program the plants right now with this technology? Thus, do you reckon you could be able to program human one day? [laughter] Cause if I understood, it's the same technology as the vaccine, right?
AK: Pretty much. That's a very good question. Yes, if we talk about, like theoretically, it is possible. I'm not sure whether you had seen the news a couple of years ago. In the scientific community, people criticize that there were some designer babies, their genome was edited, and then they were born. It was in China. So now the question is, like the, all the ethics. So if you ask me, is it possible? I would say yes, it is possible, but is it ethical? Should we do that? I can't comment really on that. Sorry.
MC: Fantastic. Any further questions? Yep.
Audience Question: Thank you. The inputs I saw, to your logic gates were temperature. How many different inputs can you process?
AK: So if I go back to my slide here. Yeah. So here I just provided an example of the temperature where we had lengthy circuit due the temperature. We had also done studies in the library. We had linked it to different chemicals, for example dexamethasone. Some people in other parts of the world, they are using some pathogens, where they can link it. So theoretically you can link it to any input signal. But that would require some optimization.
Audience Question: How deep have you your, your logic level levels gone. So in this example there's two, I saw two before. How big are your logic tables?
AK: Okay. That's so good question. So depending on... if I go at the very end here. So here I have shown the different layering of the normal gate. So two we can combine them in two different ways. And then three, so we have gone up to three. We have success variation that that it works perfectly fine. But people who are working in yeast, in bacteria, so they suggest if you go up to 5 or 6, it might still work. But beyond that, there might be some leakage or breaking of the signal. But now, like with the passage of time, you get to know more, more knowledge. So there might be things like, I mean, we can deal with those kind of issues.
Audience Question: And what's the speed on each level.
AK: So, I mean that would depend on like the...[unclear comment from audience] Yeah. So with this speed, if you take a look to the leaf data here, that it is quite robust after six hours. This is just a simple one. So we can see repression and then reverts back. But the bigger the circuit, it will take a bit more time to complete the logic operation.
Audience Question: If I wanted to make a full carry adder out of a potato, it would take maybe two days?
AK: Maybe. Maybe because here the design the protein that we are using is the Crispr protein. So then because in the lab we are working on different proteins that we are designing. So then it is just like a toolkit. So you have a problem, you have a purpose, and then depending on that you will take like this specific tool to apply to it. So I mean it is exactly the same thing. Like I mean in the beginning they just started with those few logic gates. And now we have like everything at micro level. So maybe I'm not sure whether I would be alive at the time or not, but the field would have gone like, do this kind of cutting edge technology.
Audience Question: Have you found if there's like a switching life? Like, how many times can you expose the stimuli to a gene before it stops responding?
AK: Well, I mean the thing is, so what we have done, this was done in the model organism, like Arabidopsis. We have done, it works several days. They are responsive. And then the plants, like, we have tried it in small things like 5 to 6 whole days. We have tried it in two weeks and they are quite responsive. I believe, I mean we haven't done it, but based on the work that we have done, if we don't kill it they should be responsive. So. Yeah, I mean the thing is we had to kill it for getting the results to see whether the circuit is working or not.
Audience Question: Just thinking about plants like legumes that really rely on bacteria. Is there something that you're looking at in the future, maybe, thinking about the bacteria fungi relationship between plants and other organisms?
AK: That's a very good question. In the audience there is someone, maybe you can have a chat with him. He is specifically working in the plant microbe interactions. So we are not looking into that domain, but some of the colleagues in the US and in the UK, they are working in that area. So what they are doing, so they are engineering bacteria and then checking their interaction like, I mean how they could improve the performance of the plants in the same way they have like engineered plants and then they are exposing them to those bacteria. But we are not working in that domain. Our main purpose at the moment is, because we have developed the technology now. So now we are working on the genomes to make these, it's more plants for the space exploration. So how they would taste like strawberry yogurt, those kind of like crazy ideas. We are working on it so that like it is very much easier for the astronauts so they don't have to take their food there.
MC: Wonderful. I think we've got time for one more question if there's any last ones. No, wonderful. Okay, please, thank you very much. Put your hands together.
AK: Thank you.
[Recording] Thanks for listening to the talks archive brought to you by the Western Australian Museum Boola Bardip. To listen to other episodes, go to visit.museum.wa.gov.au/episodes/conversation where you can hear a range of talks and conversations. The talks archive is recorded on Whadjuk Nyoongar Boodjar. The Western Australian Museum acknowledges and respects the traditional owners of their ancestral lands, waters and skies.
More Episodes
Writer, puppeteer, and folklorist Clare Testoni tells the story of the Moon's many faces in folk and fairy tales around the world.
Join Dr Natasha Hurley-Walker, ARC Future Fellow at Curtin University, as they explain how lunar radio waves let us explore the universe.
Join the stellar Sandra E Connelly and Dr Phil Bland in the To the Moon exhibition for a fascinating conversation about NASA's seven-billion-dollar mission to explore Earth, the Sun and beyond!
Join Richard Tonello as they discuss and debunk all things lunar conspiracy, from the flapping flag to flat earth theories, missing stars and the moon's reflectivity index.
A conversation between Dr Chantal Bourgault Du Coudray & Lucy Peach, discussing the cultural significance of the moon and how this relates to women.
Join Professor Steven Tingay to discover how the moon itself is a telescope for radio astronomers.
Join Professor Eric Howell in this talk that delves into the heart of multi-messenger astronomy, highlighting its potential to decipher the intricate mechanisms driving these cosmic phenomena.
Join Dr Robin Cook on a celestial journey exploring the mesmerising interplay of the Sun, Moon, and Earth
In this talk Professor Bland outlines the mission that Curtin is developing to find ice on the Moon in accessible locations.
Join Xavier de Kestelier, Principal and Head of Design at Hassell, to hear about Hassell’s design journey and collaboration with the European Space Agency to develop a innovative concept for a thriving settlement on the lunar surface.
Join Dr Benjamin Kaebe, an engineer, researcher and teacher, as he discusses the opportunities of a Western Australian space economy.
Professor Phil Bland provided an overview of Artemis, and what the next decade of space exploration might look like.
In this lecture, Professor Katarina Miljkovic explores the impact origin of the Moon.