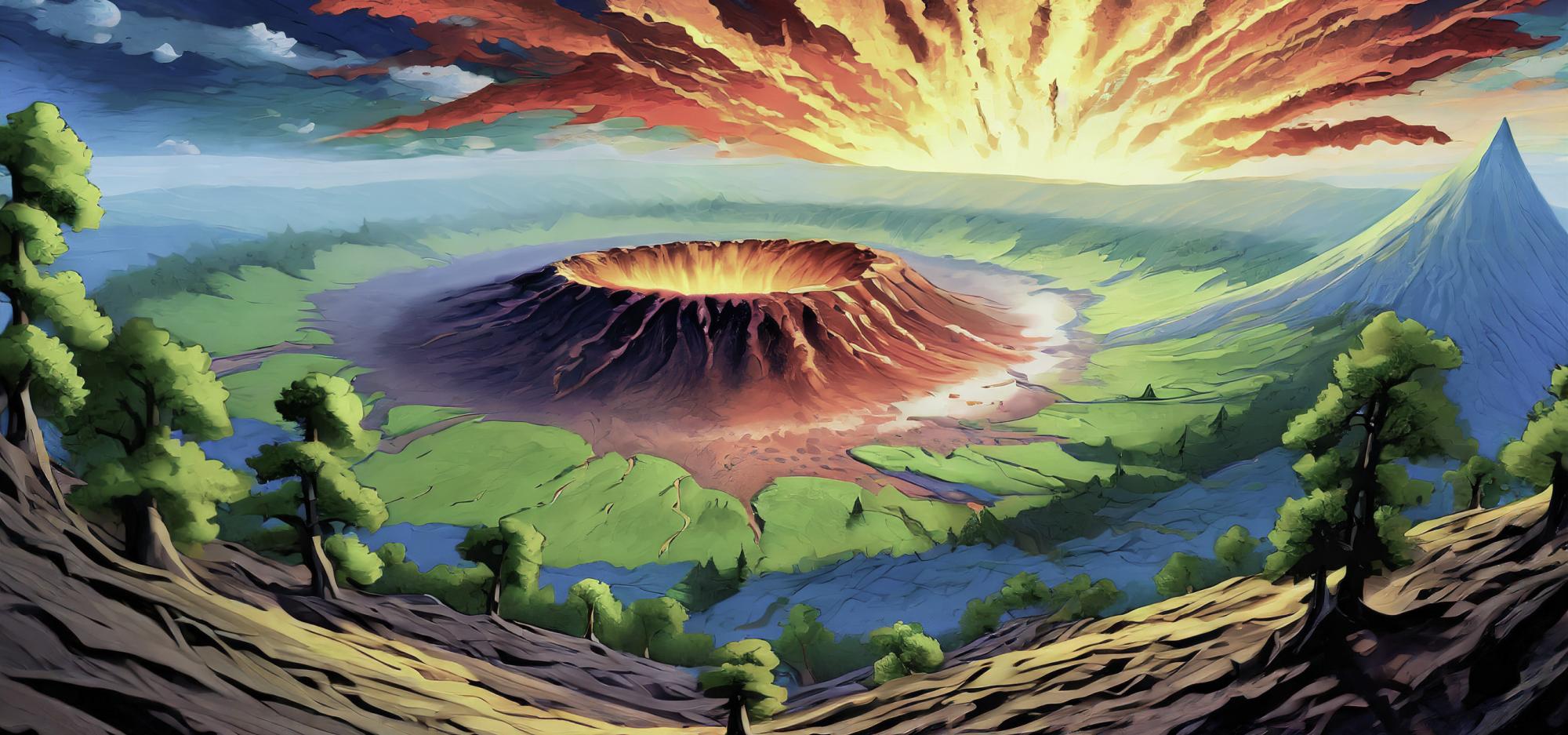
Biomarkers' Role in Mass Extinctions
Meet Professor Kliti Grice, the West Australian Scientist of the Year 2022, as she embarks on a quest to decipher the Earth's past mysteries.
Through the lens of ancient molecular fossils, biomarkers, and stable isotopes, Kliti unveils the driving forces behind five pivotal mass extinctions, including the event that wiped out non-flying dinosaurs.
In this enlightening talk, we journey through Earth's historical transformations, from species evolution and adaptation to the essential role of microbes in preserving soft tissues within extraordinary fossils. Explore diverse fossil samples, including those from the Devonian Gogo Formation, Jurassic Posidonia shale, Eocene Green River Formation, and Carboniferous Mazon Creek.
This engaging scientific expedition welcomes audiences aged 12 and above, promising a fascinating discussion that deepens your understanding of our planet's remarkable past.
-
Episode transcript
Kliti Grice: Tonight, I just want to touch on some chemistry. I’m actually a chemist, not a geologist, but I work and dabble with geological samples and I’m going to go through some of the great stuff that we’ve done at Curtin University and internationally.
So, what I first want to acknowledge is, to [acknowledge] Country, to the custodians of this land, the Whadjuk people of the Nyoongar nation and their Elders past and present. And I acknowledge and respect their continuing culture, and their importance, and [the] knowledgeable contribution they make to the life of Perth and Western Australia. And this is especially important with recent political things that have happened, so I think we still need to, we really need to endorse this.
So, also to acknowledge Arlene [Moncrieff]; and Lisa Smith, who’s our outreach coordinator at Curtin for our centre; and the WA Museum for this event. And, this work is not without a huge team of more than forty PhD students and researchers in the past twenty-five years. And obviously my own advisors through my career. So, I’ll touch on some of these people as I go along.
So, in Earth’s history there’s been five big extinction events. You’ve probably all heard about the dinosaur extinction sixty-six million years ago, but there are many other mass extinction events, which— A couple, I’ll talk about today.
There's a natural background rate to the timing and frequency of extinctions. Ten percent of species are lost every million years, approximately. Thirty percent within ten million years. Sixty-five percent on a hundred million years’ timeframe. And extinction’s good, because evolution occurs. This is a balance of extinction, the end of species and speciation, and the creation of new species. So, we wouldn’t have existed if we didn't have the dinosaur extinction, for example.
So, today I’m going to talk about the biggest extinction event, and I’m going to talk about the dinosaur extinction event, and then I’ll move on to some work which relates to fossils and how exceptionally well-preserved fossils occur in geological samples. And this relates to the first part of my talk as well.
So, just to show you that mass extinctions and climate records— This is very relevant for today because we’re going through climate change and global warming. And this is the last five hundred, fifty million years through geological time showing, in the red, the extreme hothouse conditions and in the light blue, the severe icehouse conditions. Quite a lot of these extinction events have occurred during hothouse conditions, and some in cold house conditions, but largely hothouse conditions. I just wanted to put that up just to give you some context that climate change and extreme warming has occurred in the geological past.
So, now to introduce you to fossils, the molecular fossils. On the left here we have a nice fossil prawn and, on the right, we have a molecule. Don’t worry too much about the chemistry. Just look at this as a fingerprint molecule called cholesterol. You will be familiar with cholesterol. It's present in ourselves. It’s present in the food we eat. It’s very high in prawns, for example. And cholesterol occurs in algae. And in geological samples, in rocks, which are very old, the alcohol group is lost, this OH group and a double bond, and you end up with a molecular fossil which has that carbon skeleton that resembles the cholesterol. This is called cholestane. It’s got the same number of carbon atoms.
What we also can do with such molecules which have got carbon and hydrogen, we can measure natural abundance isotopes like carbon-13 and carbon-12 and deuterium and hydrogen, which reflect the carbon cycle, the climate, the carbon dioxide used by plants, the pathway that they use to do photosynthesis and so forth. And deuterium and hydrogen reflect the source water as well. So, we can do this with these kind of molecules.
Biomarkers have been used to establish what is called the ‘tree of life’, which are these three groups called eukarya, bacteria, and archaea. We belong to the eukarya group which make, um, specific molecules. Archaea. And we’ve got bacteria. So, there are actually three domains of life that exist.
Now, to get biomarkers out of rocks. So, biomarkers all come from cell membranes, such as this of bacteria. That’s one example. Hydrocarbons in that cell membrane actually become buried in sediments and changed. The chemical structure changes a bit with the skeleton that resembles the original biomolecule. We can take these rocks, like this, grind them, extract them with common organic solvents to obtain an extract which will have several thousands of biomarkers in. And then we can analyse these by various mass spectrometry techniques and isotopic techniques.
So, that’s basically what we do. We actually crush rocks. We crush fossils, unfortunately, but we can actually get very important molecular information from these samples.
So, going to the mass extinctions. The first one I want to talk about is the Permian-Triassic extinction when life on Earth nearly died. Ninety-six percent of marine and seventy percent of terrestrial species were literally wiped out.
It was quite a good time because evolution occurred and things like this ichthyosaur, which is down here, evolved, and I’ll come back to that. We also have oil produced during the largest extinction event, particularly in the Perth basin here in Western Australia. And there are rocks which offer petroleum potential as well. So, there’s exploration that has gone on.
But this mass extinction is a tangled web of causality. There’s lots of things that lead to this event.
The Earth was significantly hotter, as I showed you on the first slide. It was a global warming event. The oceans were very stagnant and lacked oxygen. And at this time the supercontinent called Pangaea was formed. There was plate tectonic activity, a super continent formed and massive volcanism occurring.
There’s also high levels of toxic gas, hydrogen sulphide, in the oceans, which I’ll talk about today, and high amounts of carbon dioxide carrying a carbon-12 signal.
So, before I show you what happened during the P-T or some of the drivers of the Permian-Triassic extinction, I wanted to show you what occurs in the modern-day Black Sea today.
The modern-day Black Sea is an inland sea and our anthropogenic inputs like waste and— Just, all the farming products and everything have gone into the Black Sea and caused it to become anoxic, without oxygen, of a time.
The Black Sea has [an] oxygenated upper water column here with cyanobacteria, or blue-green algae, like what you get in the Swan River. And then this bottom water is completely anoxic, no oxygen. And what exists here are these sulphur bacteria, the green-green and green-brown sulphur bacteria and even purple sulphur bacteria.
These organisms use hydrogen sulphide in photosynthesis, not water like blue-green algae. And they live under strict anoxic conditions. The hydrogen sulphide is provided to them by organisms called sulphate-reducing bacteria which degrade organic matter without oxygen.
And these organisms actually live in a distinct zonation because they make very specific chlorophyll pigments which enable them to capture this longer wavelength of the sunlight. Okay? These organisms live under low oxygen, high hydrogen sulphide conditions and we refer to these as photic zone euxinic conditions.
These organisms do also occur in stromatolites, such as at Shark Bay. If you’ve been to Shark Bay, you’ll have seen maybe the stromatolites at Hamelin Pool and the microbial mats. These have cyanobacteria. They’ve got the purple sulphur bacteria. They’ve got green sulphur bacteria, as well, in these layers. But these environments do have hydrogen sulphide within them.
So, we can actually track these organisms in geological samples by looking at molecules that come from the pigments. For example, this biomarker here, molecular fossil— Don't worry too much about the structure. It has a skeleton similar to the pigments found in these green sulphur bacteria.
These bacterio chlorophyll pigments that are used in photosynthesis also have very, give rise to very unique molecules like this, from this part of the molecule. So, we find these in the rock record. We find these molecules which are from these sulphur bacteria. Okay?
So, where did we find these? This is during the largest extinction event. This is Pangaea, the super continent that was formed at that time, and these red stars are the locations where we have found evidence from the biomarkers of these green sulphur bacteria at the extinction horizon or near to the extinction horizon.
So, we can actually say that, given that this was a global warming event, given that the ocean was very limited in circulation and there [were] no ice caps at all, that it was a very sluggish circulation, a bit like you see in the Black Sea.
And it was very stratified, so you have bottom waters with little oxygen, and you have these sulphur bacteria utilising hydrogen sulphide. Hydrogen sulphide is toxic to most marine organisms and toxic to even terrestrial organisms, so we think the output has played a role in the mass extinction event of the big, biggest mass extinction of life on Earth. So, we call this killer sea water.
Driven by volcanism, we have global warming. And these conditions also occurred, and this is work that I won’t present today but, have occurred during the end-Devonian, the end-Triassic. I will touch on the end-Cretaceous. And during recovery, as well, these conditions are prolonged for millions of years. So, these are not just unique to the largest extinction event.
So, the end-Cretaceous extinction event is the one which is a bit different to most of the others, in that this is linked to a meteorite impact. This is when the non-avian dinosaurs were wiped out. Our birds today are the avian dinosaurs as such.
And this was the work of Bettina Schaefer for her PhD. Seventy-five percent of plant and animal species became extinct. Some forams and calcareous organisms and even plants on land were significantly affected.
This was caused by an asteroid. The asteroid hit the Gulf of Mexico and this led to massive amounts of aerosols, and soot, and basically no sunlight, and therefore algae or cyanobacteria and phytoplankton declined in the oceans as well, at this time. So, it was quite catastrophic. And that provides food for other organisms, so organisms depending on these phytoplankton also got wiped out.
So, the end-Cretaceous extinction event was driven by an asteroid which hit the Gulf of Mexico sixty-six million years ago, which led to a one hundred and eighty kilometre-wide crater being formed. It’s not the largest crater on Earth but I think it’s the second largest one and the asteroid impact was the equivalent of the energy of several million nuclear weapons detonating simultaneously. So, it was [a] massive impact.
We were very fortunate to be part of a major international ocean drilling program. This is my colleague at the front here, Marco Coolen, who actually went on the expedition in the Gulf of Mexico to help drill this core through the Chicxulub Crater. The Chicxulub is the crater where the asteroid hit the Gulf of Mexico. And they drilled through the crater and obtained a core of 506 to 1335 metres below sea floor.
This was done with this, [Liftboat] Myrtle. What was achieved was forty-eight to sixty-six million years’ timeframe, which spans the extinction, which is called the K-Pg which is shown here. It’s the Cretaceous-Palaeogene extinction. This is an extinction horizon, okay?
What’s interesting is that this core, the bottom part of the core, has this granitic basement. Then this blue part is actually a tsunami layer which is very deep and was accumulated within a day.
So, you can see that’s [a] massive amount of sediment from six hundred metres below sea floor to seven hundred metres below sea floor. And then these sediments above [are] the normal sediments after the extinction. The core itself, as I said, has this granitic basement. I’ll show you some of the nice kitchen benchtops that you would find at home.
And then you have the tsunami layer here, and we have sediments above that. So, this is the sorts of material that we were working on, or specifically Bettina was working on.
Now, we’ve been able to track the tsunami, which occurred one day after impact, using molecules in those rocks which is quite an achievement. And the two molecules, without worrying about the chemistry again, just see these as sort of fingerprints. One is called perylene, which comes from fungi that degrade wood in situ, in sediments. It’s a black pigment called perylene quinones. And this second one is called benzo[a]pyrene which is indicative of fire.
So, we can track these two molecules through time by looking at their relative abundance. What we do know about perylene, though, is that it’s actually formed in situ in sediments as sediments accumulate. And therefore, deeper sediments generally have a higher proportion of perylene as accumulation occurs, and that you generally find in deeper sediments [that] you’ve got more perylene. So, that’s a typical kind of profile you might find in normal sediments.
What we see in the Chicxulub Crater, if we plot these two molecules, just the ratio of perylene over benzyl[a]pyrene— So, we’re plotting the fungal degrading marker over the combustion marker. We see almost an opposite profile in this, what is the tsunami layer here. This is the tsunami layer within one day after impact.
So, that shows that the perylene – this is the height of the tsunami, is high here. And then it starts to swing back when normal sedimentation occurs, so when the tsunami stops, and you have smaller little tsunamis. You can see it kind of increasing.
So, this is tracking the waves of the tsunami one day after impact, and this means that all the terrestrial material which would have looked like this has been uplifted and overturned and transported into the crater and then started to settle. That’s within two to three years to thirty thousand years when settling started to occur. This is within a day. So, the height of the tsunami would have been the same height as the BHP Billiton building in Perth. So, pretty massive.
So, with the Chicxulub, without showing you all the data, we have published a lot on this and, in particular, Bettina Schaefer has done [an] enormous amount of work looking at the Chicxulub.
But this is a nice summary slide of what we’ve found using the molecules. So, we can see markers of the tsunami. I don’t know if this is working. Can you see that? No, it’s gone dead I think. Oh no, it’s come back again.
So, the tsunami we can see with molecular markers. We can see combustion of forest fire markers. We actually track compounds that come from that. We do see indicators of cyanobacteria and what’s interesting is, these are the phytoplankton that use sunlight. They actually returned quite quickly, within years to thirty thousand years after impact.
So, they recovered quite quickly after that darkening period, and then stable conditions occurred much later. And we do see evidence of these euxinic conditions with these sulphur bacteria as well that we saw in the Permian-Triassic extinction event.
So, what we find very important is using molecules and, looking at these kinds of profiles in deep time, that we get glimpses of the past. We can see evidence of these low oxygen conditions in the past— Let me just see if I can get that to work. Yep. See evidence of— We can even see evidence of acidification, low oxygen conditions from molecules. We can see the evidence of the carbon cycle. We can see climate warming.
What’s interesting is that, in the past, the CO2 is a different source of the CO2 today which is being driven by anthropogenic activity since the industrial revolution. But we’re seeing similar things appearing, like low oxygen conditions on our shorelines. We’re seeing coral bleaching. We’re seeing algal blooms and decay causing anoxic conditions. We have ocean warming, etcetera, etcetera. But the carbon dioxide is of a different origin.
And this carbon dioxide is accelerating at a much greater rate than in the geological past. So, we do see the past is key to the present and the future, and this can be done from molecular biomarkers.
Now, moving to the second part, which is the really new stuff that we have been doing at Curtin University for about ten years now, is fossil preservation. You will be familiar with normal fossil preservation here where the original material is completely replaced by minerals. We can have things like selective preservation where certain polymers, such as—waxes on leaves are actually polymers—can actually survive degradation and be preserved. And you can have exceptional preservation when original material avoids full decay, and you get soft tissue and you can have even molecules still preserved in those tissues. And I’ll show you some examples of that.
One of the best ways, we think, of preserving soft tissue are the— One of the types of fossils that preserve soft tissue are those that occur in concretions. Concretions are like limestone mounds. They vary in size, from metres to centimetres, and they can contain really well-preserved fossils. So, an example here is a concretion, and there’s a fossil inside there, really well preserved. And there may well be molecules as well, preserved in the concretions.
And I’ll come back to how quickly these are actually formed a bit later, but these concretions do occur in Western Australia in the Canning Basin. In the northwest of Western Australia, we’ve got the Canning Basin, which is our Great Barrier Reef from the Devonian, three hundred and eighty million years ago, and in these concretions you have these beautifully preserved 3D fish.
You might have seen the Gogo fish in the Museum here that was found by John Long and Kate Trinajstic. And you see original bone with histological detail and you can even see embryos and things like that preserved inside these concretions, embryos of fish.
So, this is one of those concretions. I always present this because this is the start of the work that we did, and it’s like an evolutionary story as we go along. So, this is a concretion from the Canning Basin that is three hundred and eighty million years old. It doesn’t look very much. It looks a bit... It doesn’t look like anything. Apparently, it’s an invertebrate but the palaeontologist didn’t know what kind of invertebrate.
It’s called ‘Mushier’ because it does look messy or mushy. <laughs> I don’t know. But this was taken for geochemistry studies and the soft— This has soft tissue. This grey stuff is, and all this white stuff, is soft tissue. This is the limestone material surrounding it. This is the outside part.
So, this was work done by Ines Melendez, who now works at Woodside. She looked at this fossil, she separated the fossil from the matrix, this limestone matrix and, without being a geochemist, I can just say that these fingerprints you see on the right for the fossil and the limestone matrix look quite different. And they are different. Those are the chemical signals of all the molecules in the fossil and the matrix.
What’s intriguing is that this fossil contains a lot of C-27 cholestane. So, if you remember at the beginning, I said cholesterol gives cholestane. Well, here’s our cholestane marker.
What we could do— We also found, which was intriguing, just in the soft tissue, not in the limestone was our biomarker of green sulphur bacteria. Kind of pops up and it’s indicative of green sulphur bacteria. What's that doing there? Well, I’ll come back to that as well.
And the concretion has molecules which are from the bacteria that reduce sulphate. What is also present as steroids, as I said, dominated by 27 steroids. Um, so from cholesterol. And Ines was able to work out what percentages of the steroids in this soft tissue and that was concordant with a very high percentage which are found in crustaceans, so, which are the prawn that you eat.
So, lots of cholesterol, and we could say that this invertebrate was a crustacean. It was not a mollusc because it didn't have a shell but it was definitely a crustacean.
And we were also able to get the isotopic value for the diet of this crustacean. So, it’s a prawn, basically. A big prawn. Sort of, quite a big prawn. <laughs> Probably one of the biggest you’ll ever see.
And what was also in the soft tissue were seventy different steroidal compounds which come from cholesterol itself. When an organism dies all of that cholesterol is degraded into many different molecules. It goes through many different transformations, and we found all those transformations in this soft tissue.
So, we found the diagenetic continuum of cholesterol to cholestane in the one sample, which was a first ever. And sterols in particular, intact sterols like cholesterol, a whole suite of different rearranged products of cholesterol and all these intermediates.
But how did this— What happened to this organism to get into this state? Well, it could have died because of toxic hydrogen sulphide. Like, was [it] an extinction event at this time, the Devonian extinction?
However, this little prawn was happily eating the phytoplankton. It had cholesterol in its body taken from its algal diet. Probably died because of H2S, like, being toxic.
But the soft tissue had this green sulphur bacteria present. The markers of the green sulphur bacteria ... photosynthesis with hydrogen sulphide, they’ve probably built a biofilm around the prawn and that actually helped to preserve the soft tissue. On one hand, it might kill the organism, but on the other hand it helps to preserve the soft tissue. And then the organism sank and the sulphate-reducing bacteria degraded the organic matter further and built a concretion of limestone around the decaying carcass as alkalinity dropped.
The next slides relate now to an ichthyosaur. This is quite spectacular. This is an ichthyosaur which occurs in a Jurassic concretion which is one hundred and eighty-three million years old. This is the work of Chloe Plet.
We have calcite. We have quartz. We have the bone, and when you treat the bone with light acetic acid you see collagen fibres preserved in this hundred and eighty-three million year old bone. We found red blood cells, as well, inside the bone from the ichthyosaur.
These are a bit smaller than the modern. We also find cholesterol intact in the bone, as well, and the cholesterol is indicative of the diet of the ichthyosaur.
There are these strange isotopic values. For those who do isotopes, the cholesterol is in the bone that— The ethyl cholesterol is the diet of the ichthyosaur, at the base of the food chain. So, you are what you eat, plus one and a half per mil per trophic level, for the people who do food chain research. So, there’s that offset as well. And we find all these steroid compounds as well. This entire diagenetic continuum in this bone. And we find green sulphur bacteria as well, as we found in the Devonian concretion.
But what's interesting is, the ichthyosaur evolved following the Permian-Triassic extinction when the conditions in the atmosphere were low in oxygen. We have the dietary sterol, we have preservation, maybe biofilm as well around this bone. And we have collagen fibres and small red blood cells.
The red blood cells are smaller than most modern— We think that is because of the low oxygen conditions and it had to make small size red blood cells to efficiently transport oxygen around the body. A bit like when animals that live at high altitude, they naturally make smaller red blood cells to increase the surface to volume ratio. And we find remains of haemoglobin as well.
The last few minutes of my talk— I'm conscious of the time. I’ll just keep going for a few more minutes. It’s related to Mazon Creek. This is the work of Madison Tripp, who’s in the audience, who finished her PhD last year. And Mazon Creek is three hundred and six million years old approximately. It’s near Chicago and it’s a deltaic system. And there’s concretions in this environment as well.
This is not a marine setting. It’s very different to the two examples I’ve shown you but there are, inside these concretions, many, many different plants and animal species preserved.
There's Madison. There’s all these reactions that occur, which I won’t go into. But this is an example of coprolites. Does anyone know what coprolites are?
<audience murmur>
Well, yes. Fossilised poo! <laughs> And these occur in iron carbonate, not limestone. These are called siderite concretions, and these coprolites have lots of nice minerals.
But what's interesting is that these coprolites are actually, if you look at the chemistry, dominated by— We have here the fossil on the top, so the fossil coprolite dominated by C-27 steroids, and then the matrix surrounding this has a different composition again. Has a mixture of sources.
So, these are very, not very well matured. And then ninety-nine percent are basically C-27 steroids in one and ninety-three percent are steroids in the other. That's the matrix there. What we can say about this is that, based on these steroids and the abundance of these steroids, that the diet of these coprolites must have been a carnivore.
Okay, so we can actually look at the steroid distributions and say, well, that was a carnivore that produced that. You can do fancy stuff with Raman spectroscopy, looking at the polymeric material and the functional groups within in these kinds of coprolites.
And I’ll just, without showing you— I’ll just show you the plot of the data. So, these are—let me get there—these are carnivores. This is based on lots of chemical markers. I won’t go into the details. We can distinguish herbivores from the polymeric material. And then you can see the coprolites here, these two, were ... to the carnivores. And they also contain some shell fragments. But that also is consistent— The biopolymeric material that was measured by Raman was consistent with a carnivorous diet.
So, what we’re trying to do is complete the story of fossilisation at Curtin and with our international colleagues. By looking at these concretions, which we know are actually formed within days to weeks after the animal has died or the organism has died. And that allows rapid encapsulation of organic remains, which is why we have this enormous opportunity to look at molecules, intact, in these very well-preserved concretions.
So, we’re doing that on a geological, in a geological sense, but we’re also doing it in a microbiological sense where laboratory experiments have been, are being performed where you can take microbial mats. Like this microbial mat, which I showed you at the very beginning, the one on the right here from Shark Bay which has cyanobacteria, and it has got that gluey green sulphur bacteria which built the biofilm around the Devonian prawn. And we’re actually using this material, growing the mats in the lab, and inserting different types of leaves and insects and bones, and mimicking fossilisation.
So, it just gives you a snapshot, and I’d just like to finish there because I’m conscious of time. But I hope you found it a little bit interesting, and I want to thank you for being here tonight. Thank you.
<applause>
Arlene Moncrieff: Wow. That wasn’t long at all compared to the timeframes you’ve been talking about, Cliti, so you did really well, actually. Are you happy to take some questions?
Kliti Grice: <laughs> Yep, sure.
Arlene Moncrieff: So, if you’ve got some questions, I am getting old so if you’re over there next, give me a minute to get across to you.
Audience member 1: Just looking at that cryo date[?] at the Yucatán Peninsula. I once watched a documentary that kind of made a point that if that asteroid, the ten-kilometre diameter asteroid, had hit Earth at a different time— Say, it hit the ocean where it was deeper. Would the impact have been just as devastating as compared to where it hit in the shallower parts of the Yucatán Peninsula? And would it have caused that mass extinction?
Kliti Grice: <laughs> Good question. Do you need me to just—
Arlene Moncrieff: Yes, please, because I can’t answer it.
<audience laughs>
Kliti Grice: <laughs> Do you want me to use the microphone?
Marco Coolen: <inaudible>
Arlene Moncrieff: Hang on. Can you start again, please.
Kliti Grice: I was just going to answer it but... <laughs>
Marco Coolen: It actually wasn’t shallow. It’s shallower now.
Kliti Grice: Yep.
Marco Coolen: But it was like, six hundred metres deep at that point. Because, you know, over the sixty-five million years there’s lots of sedimentation. So now it’s, yeah, only seventy metres deep there but it was maybe a kilometre deep back then.
Kliti Grice: It would have been different in the deeper ocean for sure. Um, so. I don’t know if, maybe it would it have been less of an impact. Because it hit a carbonate platform. It hit solid rock sort of six hundred metres deep but it [was] still relatively shallow. So, the dynamics would have been very different if it was in the deeper part of the ocean, for sure. So, it’s like dropping a pebble into a pond and you know, the different effects you have when you drop a rock onto something or into water. It will have a different effect. But it might have been less of effect, I think.
Marco Coolen: <inaudible>
Arlene Moncrieff: Okay. Next question at the back. Can we move on?
Audience member 2: Sorry, it was more of a— Yeah, I’ll hold that. It’s just a little add on to exactly what Kliti was saying and the fact that it hit a carbonate platform. Remember, a lot of the minerals there were, like, volatised and turned to gases immediately and that added to, like, the toxicity. If it had hit the deep ocean, sure, there would have been a much bigger wave, but it wouldn’t have hit such a volatile area of minerals. They actually, all became gases and got thrown up, so the carbonate platform was actually— It would have still been very destructive, but the carbonate platform was really crucial, as Kliti was saying, so quite ... toxicity as well.
Kliti Grice: Yep.
Arlene Moncrieff: Was that the same thing? Because I think you were wiggling your hand before. Anybody else? Does that answer your question? Kind of, eh?
Audience member 1: I didn’t realise it was ... deep.
Arlene Moncrieff: Anybody else? Any more questions?
Marco Coolen: <inaudible>
Arlene Moncrieff: Marco, can I get you to speak into the microphone? Otherwise it doesn’t get recorded for the people not here. No one?
<audience inaudible>
Audience member 3: I saw the ichthyosaur fossil and the interesting thing for me is— Now, that survived the Permian extinction, but the ichthyosaurs would have already been fully aquatic by then, I’m thinking. Because we don’t have transitional fossils like we do with whales, you know, where we have terrestrial slash aquatic forms of ichthyosaurs. Now if ninety-six percent of marine species died during the Permian extinction, how do you think the ichthyosaur, kind of, like, survived and remained in the four percent of remaining species?
Kliti Grice: It didn’t. It actually evolved after the Permian— It didn’t actually survive. It actually was one of the evolutionary species from the extinction itself. Sorry if I didn’t make that clear in my talk but that’s— Yep.
Audience member 3: So, one—
Arlene Moncrieff: Up-bup-bup!
<audience laughs>
Arlene Moncrief: I’m on to you.
Audience member 3: So, to be able to evolve, surely it would had to have survived the extinction?
Kliti Grice: No, when I talk about evolution, I mean new species of evolution, and so it’s, therefore— So, it’s not recovering. <sigh> How do I— Maybe Steve can answer. It’s to do with— It to do with after the event. It wasn’t there before.
Audience member 3: It started from scratch?
Kliti Grice: Yeah, it started from— Steve.
Stephen Poropat: Essentially, you have— The ancestor of ichthyosaurs has survived the Permian-Triassic extinction. Whether or not it’s aquatic already, we don’t know.
Kliti Grice: Yep.
Stephen Poropat: If it is, it’s probably freshwater because otherwise there’s a fair chance it’s probably gone out with the Permian-Triassicextinction, but then they’ve become aquatic and very big, very fast after the Permian-Triassic extinction. Something like nine metres long about three to five million years after the impact, after the extinction event. So, what they do very well, very fast after that Permian-Triassic boundary. But they weren’t—
Kliti Grice: They weren’t there.
Stephen Poropat: They weren’t in the oceans, at least not commonly, before that. Whether or not they’d even evolved as ichthyosaurs at all before the Permian is still not sure. We’ve got no fossil for the Permian of ichthyosaurs or their predecessors. I mean, beyond other reptiles from which they might have come.
Kliti Grice: Thanks, Steve.
Audience member 3: So, just quickly— Sorry to be a pain—
Arlene Moncrieff: You’re not a pain. It’s all learning.
Audience member 3: So, something survived, and it evolved from that.
Kliti Grice: Yep, something primitive. But we don’t know what it evolved from.
Stephen Poropat: So, I guess, like, if we looked at reptiles today, you’ve got two sort of major branches that are, well, not even in the form of all reptiles now but amniotes. So, organisms with an amniotic egg. So, that’s today’s mammals, reptiles, birds, that’s it. Those, the shared ancestry of amniotes goes before the Permian-Triassic extinction.
So, they were already around and they’ve split into what would become mammals and what would become other reptiles and birds and dinosaurs. That split occurs before the Permian-Triassic boundary. It’s after that when, basically, when sort of the brakes get pulled off on these animals and they just explode and then they radiate into all these different species.
Arlene Moncrieff: We’ve got a question over here. You still happy to keep answering them, Kliti, and others?
Audience member 4: So, Kliti, I was just wondering. You're a chemist primarily. Was it fossils that interested you at first, or did you get into this specialisation further into your career?
Kliti Grice: Oh, I did geology at high school and I was one of the very few people who did geology and I had to go to a special college to do that because there was— I think I was one of two people in my school that wanted to do geology. So, I always had that interest there and the analytical techniques I learned as a chemist, and I think it's that natural interest of the natural world and earth sciences but using my strength of chemistry to study the past.
So, yeah. Not many people do this. Lot of chemists look at modern material, do different work. Going into deep time is quite hard, I would say. It’s more challenging, but I think it’s fabulous to be able to take a rock, look at a series of rocks, and to scale that up to a mass extinction event, you know, over millions of years from those tiny molecules you can’t see. You’ve got to analyse them on a trace analytical level. That’s what I find really exciting, to be honest. <laughs>
Audience member 5: I’m glad you mentioned you were a geologist first! Just looking at the Chicxulub crater again on the Yucatán Peninsula. Now, one thing that was unique in that area, geologically, is the sink holes, the cenotes, in the like, northwest part of that picture. Why are they unique and how do they form after the Chicxulub impact?
Kliti Grice: Ooh, I don’t know a thing about sinkholes. Cenotes.
<audience murmurs>
Kliti Grice: We’ve got a geologist in the audience.
Audience member 6: So, can I ask, have you found anything so far that you don’t have an answer for and you’re looking forward to keep on unravelling later?
Kliti Grice: Oh, lots of things! <laughs> Just like—
Audience member 6: Do you have a favourite that you want to—
Kliti Grice: A favourite? I’m just looking at my group.
Audience member 6: Is it better than the sinkhole question?
<audience laughs>
Kliti Grice: A favourite. I haven’t, I don’t think I— I find everything intriguing that we look at. I haven’t got— It’s just really hard to say.
I guess, the pterosaur which is on the slide there, is one I’d really like to look at and we have, hopefully, an opportunity—I’m looking at Steve—to look at the little thing on its head, whatever it’s called—the crest—in terms of fossil preservation in the Santana formation. And we are going to have an opportunity, we are working with Brazil's Rio museum and we’re going to be working on the Santana formation. And I think that is probably the best-preserved deposit, deposits in the world.
We do have the Green River but they’re slightly different. But the Santana formation has these huge nodules which have, do have pterosaurs preserved inside. And I think that would be the icing on the cake, is to be able to work on a dinosaur in a concretion because there you will be able to get probably the most spectacular molecular information ever, to be quite honest. That would be my, I guess—
Audience member: ... come back to life?
Kliti Grice: <laughs> Yeah.
Arlene Moncrieff: Any other questions?
Audience member 7: Just a quick one. A quick question here. Sorry. I’m just interested in the analogy between past extinctions, major events and now. So, with your markers, you’ve been able to sort of obviously show that they’re related to mass extinctions. Is there an absolute sort of quantification that tells you whether there’s like a, how that relates to conditions today? I mean, you’ve got one example of the Black Sea, I suppose.
Kliti Grice: Yeah, the other example would be the— I actually have a, I might even have a slide just to— This might be of interest. I put this at the end, and it wasn’t because— I took it out earlier. One of the biggest global warming events is across, is in the Eocene. It’s called the Palaeocene-Eocene thermal maximum, just after the dinosaur extinction. And that is the closest to our modern global warming.
And you can actually look at molecules in rocks that span that interval to reconstruct ocean temperature and temperature changes. And that is, that’s probably the best analogue to your question is to look at that event, which is more recent and has a very similar— The continents are very similar to today. But the sources of carbon today—you can see it on this slide—shows you the temperature rise and the modern and the gas, the duration of gas release.
This is the PETM on the bottom, which is much steadier, slower state of emissions of 1.77 hectograms of carbon per year, as opposed to the modern, which is massive. So, as when I said at the beginning that carbon dioxide emissions are much greater, greater speed now than they were in the past. But we can look at those intervals using molecules to reconstruct the temperature of the oceans and temperature during that event, which tells us a lot about the modern changes.
The modern changes are so rapid. I don’t know. It’s like, yeah. It's hard to correlate what's happening right now in sediments because it’s— You need time to accumulate, you know, to look at things over thousands or millions of years and we— Yep. I don’t know if that kind of answers your question.
Audience member 7: Well, I think it does. ... points you in the direction, doesn’t it.
Kliti Grice: So, you can actually use isotopes to measure those temperature changes, then, in that interval of the last, basically, the last global warming event which is comparable to today.
Arlene Moncrieff: Could I get you please— Thank you, Kliti.
<audience applause>
More Episodes
A compelling discussion shedding light on the distinctive narratives of those openly embracing their HIV-positive status and what this has meant historically and in today's world.
Join a panel of featured young LGBTQIA+ writers and storytellers as they delve into the fantastical realms and real-world struggles of the exciting new anthology, An Unexpected Party.
Dr Pilar Kasat, CEO of Regional Arts WA, will share her career journey as the featured guest in the second instalment of My Australia Story conversation series.
Explore Australia's dinosaur legacy with Dr Stephen Poropat as we better understand the science behind the scenes of Australian palaeontology!
As we inevitably move towards cleaner, more sustainable forms of energy, how will a shift away from traditional mining practices transform this economy? A panel of experts explore the implications of this shift with a specific focus on how we see ourselves in a world that increasingly demands environmental responsibility.
Join Megan Krakouer, a prominent Aboriginal leader, as she discusses the nuances of her surprising change of heart on the Voice to Parliament — just a week before the referendum.
Join host Michael Mills in a captivating live recording of the Palaeo Jam podcast during National Science Week!
As AI rapidly evolves, join our panel of experts in an exploration of these complex issues, including how we can harness its power while ensuring responsible and ethical use before we're outrun by its speed.
Do you know how and why your teeth do what they do? Find out all there is to know about teeth sharpening.
Decoding the language of our written and oral histories in Australia.
Join us for an insightful talk with the esteemed Professor Henry Skerritt, as he takes us on a journey exploring the captivating art of Jdewat/Ballandong artist Meeyakba Shane Pickett.
No, it’s not a card game but a community initiative helping us further our knowledge of a group of endemic land snails Bothriembryon affectionately called ‘Boths’.
Delve into the way Aboriginal industry professionals are shifting representations, decolonising the media space and creating visibility for Aboriginal people in the industry.
Exploring the debate surrounding the establishment of an independent, representative advisory body for First Nations people.
The first in a series of My Australia Story conversations, meet Dr. Sandy Chong (BCom Management and Marketing, 1996, PhD Information Systems, 2003).
Aristeidis Voulgaris sharesstories from his eclipse chasing travels and discover the complex instruments and tools used in solar astronomy.
Join a panel of experts and community as they investigate the nature of queer bodies, and queer sexuality, in public space.
Join historian Toner Stevenson and astronomer Melissa Hulbert, as they share their tips on how to salute the sun during this eclipse!
Simon Miraudo and Tristan Fidler from RTRFM’s ‘Movie Squad’ are joined by Chelsey O'Brien, Curator at ACMI, as they review and discuss Alice’s Adventures in Wonderland as a foundation for contemporary storytelling in film.
In this one-off Perth Design Week talk, the Museum celebrates the remarkable women in architecture and film in a panel discussion, recorded as a part of its recent award-winning film screening.
Join world expert Dr Chris Mah from the Smithsonian Institution as he recounts his encounters with some of the most unusual creatures on the planet.